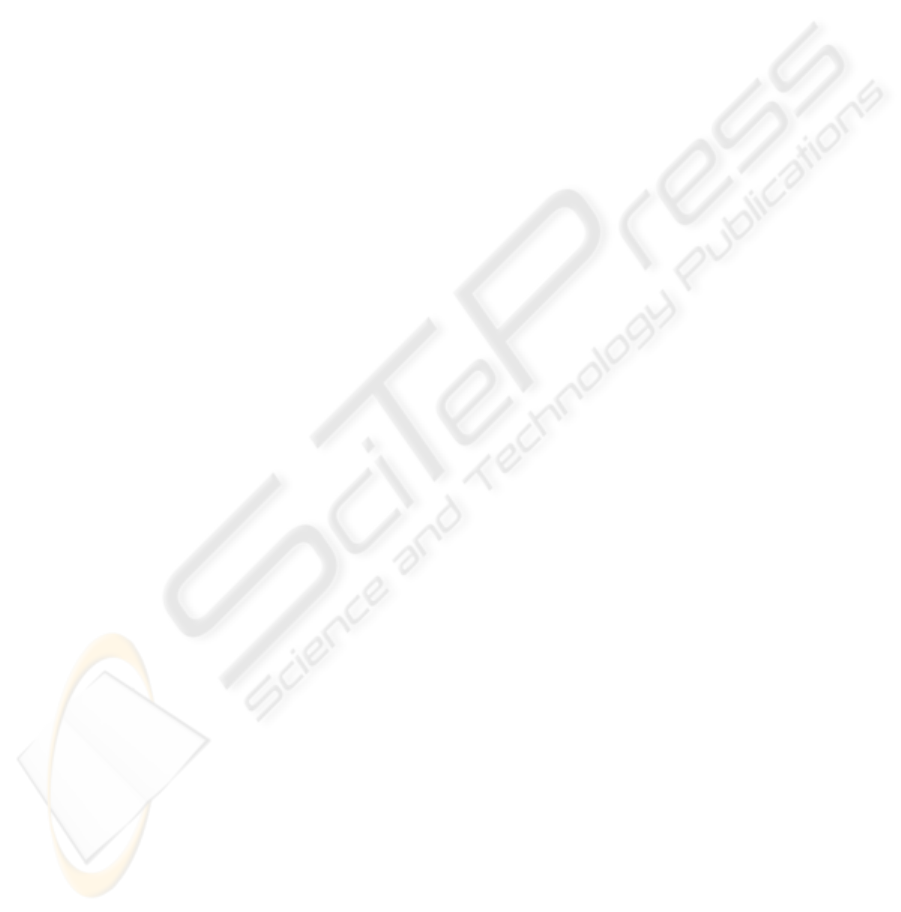
Because the sensor technique of the continuous beat-to-beat measurement method is
not at all influencing on the persons attention, the continuous model based blood
pressure measurement proves as valuable additional indicator for the psychological
conditions and the stress levels of the test participants. Although intraindividual dif-
ferences in the reactions to special situations can be observed, in general it can be
quoted that all participants show similar results. Due to personalized models our
blood pressure determination method is sensitive even to small signal changes.
Acknowledgements
The authors thank Dr. Jürgen Locher and Regina Sprenger from L-LAB Paderborn for
providing the Vienna Test System and making test drives with the “Nightdriver”
Simulator possible. We are also grateful to Prof. Dr.-Ing. Bernd Henning and Dipl.-
Ing. Stefan Schlößer from the Institute of Electrical Measurement of the University of
Paderborn and to Prof. E. Trowitzsch, University of Witten-Herdecke/Vestic Chil-
drens Hospital, Datteln, for helpful discussions.
References
1. Finapres Medical Systems, "Datasheet Finometer and Portapres",
http://www.finapres.com/index.php?pid=4200, 2002.
2. Medwave, "Vasotrac APM 2005A System Features and Specifications", 2005,
http://www.pmsinstruments.co.uk/vasotrac.htm
3. D. Barschdorff, M.Erig, "Kontinuierliche Blutdruckbestimmung während des Belastungs-
EKG", Biomedizinische Technik, vol. 43, no. 3, pp. 34-39, 1998.
4. D. Barschdorff, M. Erig, E. Trowitsch, "Noninvasive continious blood pressure determina-
tion", XVI IMEKO World Congress, Proceedings VII, Wien, 25.-28. Sep. 2000.
5. D. Barschdorff, C. Bauch, "High resolution beat-to-beat measurement of systolic blood
pressure using personalized models ", Proc. 10 th IMEKO TC 10 Int. Conf. on Techn. Di-
agnostics, Budapest, 9.-10. June 2005.
6. D. Barschdorff, C. Bauch, "Application of high resolutionblood pressure measurement to
stress and traffic situations "Proc. 10
th
IMEKO TC 10 Int. Conf. on Techn. Diagnostics,
Budapest, 9.-10. June 2005.
7. R. Busse, "Kreislaufphysiologie", Thieme, Stuttgart, 1982.
8. R.F. Schmidt, G. Thews, "Physiologie des Menschen", Springer, 1997.
9. P. Elter, "Methoden und Systeme zur nichtinvasiven, kontinuierlichen und belastungsfreien
Blutdruckmessung", Diss., Univ. Karlsruhe, pp. 1-162, 2001.
10. Roche "Lexikon Medizin: Schellong-Test", 2003,
http://www.gesundheit.de/roche/ro32500/r34385.000.html
11. R. Schandry, "Psychophysiologie", Urban & Schwarzenberg, 1981.
12. K. Rogge, "Physiologische Psychologie", Urban & Schwarzenberg, 1981.
13. G. Schuhfried: "Vienna Test System", http://www.schuhfried.at/eng/wts/wts_index.htm
134