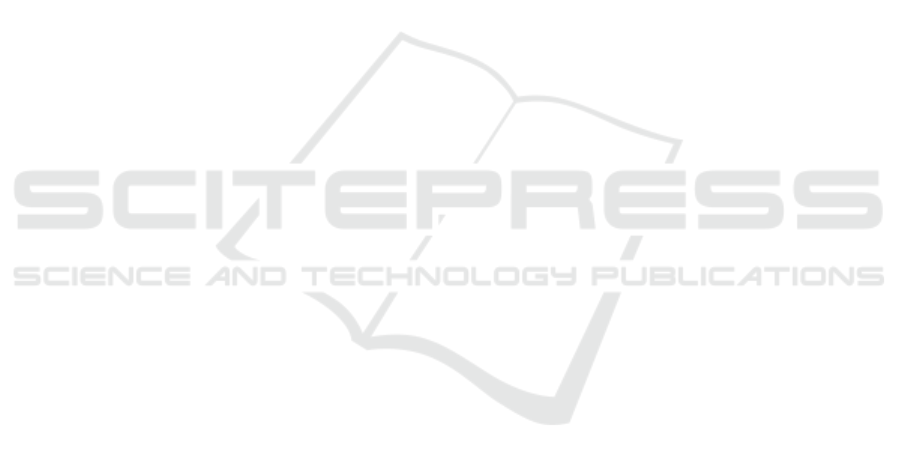
reaction measures. In Proceedings of the Human Fac-
tors and Ergonomics Society Annual Meeting (Vol.
59, No. 1, pp. 647-651). Sage CA: Los Angeles, CA:
SAGE Publications.
Chandra, S., Sharma, G., Verma, K. L., Mittal, A., & Jha,
D. (2015). EEG based cognitive workload classifi-
cation during NASA MATB-II multitasking. Interna-
tional Journal of Cognitive Research in Science, En-
gineering and Education, 3(1).
Daviaux, Y., Bey, C., Arsac, L., Morellec, O., & Lini,
S. (2019). Feedback on the use of MATB-II task for
modeling of cognitive control levels through psycho-
physiological biosignals. In 20th International Sym-
posium on Aviation Psychology (p. 205).
Falah, J., Khan, S., Alfalah, T., Alfalah, S. F., Chan, W.,
Harrison, D. K., & Charissis, V. (2014, August). Vir-
tual Reality medical training system for anatomy ed-
ucation. In 2014 Science and information conference
(pp. 752-758). IEEE.
Galante, F., Bracco, F., Chiorri, C., Pariota, L., Biggero, L.,
& Bifulco, G. N. (2018). Validity of mental workload
measures in a driving simulation environment. Journal
of Advanced Transportation, 2018.
Geszten, D., Koml
´
odi, A., Hercegfi, K., H
´
amornik, B.,
Young, A., K
¨
oles, M., & Lutters, W. G. (2018).
A content-analysis approach for exploring usability
problems in a collaborative virtual environment.
Hassandra, M., Galanis, E., Hatzigeorgiadis, A., Goudas,
M., Mouzakidis, C., Karathanasi, E. M., & Theodor-
akis, Y. (2021). A virtual reality app for physical and
cognitive training of older people with mild cognitive
impairment: mixed methods feasibility study. JMIR
serious games, 9(1), e24170.
Kim, H. G., Cheon, E. J., Bai, D. S., Lee, Y. H., & Koo,
B. H. (2018). Stress and heart rate variability: a meta-
analysis and review of the literature. Psychiatry inves-
tigation, 15(3), 235.
Kennedy, L., & Parker, S. H. (2017, June). Making MATB-
II medical: pilot testing results to determine a novel
lab-based, stress-inducing task. In Proceedings of the
international symposium on human factors and er-
gonomics in health care (Vol. 6, No. 1, pp. 201-208).
Sage CA: Los Angeles, CA: SAGE Publications.
Kr
¨
uger, J. K., & Suchan, B. (2016). You should be the spe-
cialist! Weak mental rotation performance in aviation
security screeners–Reduced performance level in avi-
ation security with no gender effect. Frontiers in Psy-
chology, 7, 333.
Labedan, P., Darodes-De-Tailly, N., Dehais, F., &
Peysakhovich, V. (2021). Virtual Reality for Pilot
Training: Study of Cardiac Activity. In VISIGRAPP
(2: HUCAPP) (pp. 81-88).
Lele, A. (2013). Virtual reality and its military utility. Jour-
nal of Ambient Intelligence and Humanized Comput-
ing, 4(1), 17-26.
Letondal, C., Vinot, J. L., Pauchet, S., Boussiron, C., Rey,
S., Becquet, V., & Lavenir, C. (2018, March). Be-
ing in the sky: Framing tangible and embodied in-
teraction for future airliner cockpits. In Proceedings
of the Twelfth International Conference on Tangible,
Embedded, and Embodied Interaction (pp. 656-666).
Liu, S., & Nam, C. S. (2018). Quantitative modeling of user
performance in multitasking environments. Comput-
ers in Human Behavior, 84, 130-140, p. 83.
Luong, T., Martin, N., Raison, A., Argelaguet, F., Diver-
rez, J. M., & L
´
ecuyer, A. (2020, November). To-
wards Real-Time Recognition of Users Mental Work-
load Using Integrated Physiological Sensors Into a
VR HMD. In 2020 IEEE International Symposium
on Mixed and Augmented Reality (ISMAR) (pp. 425-
437). IEEE.
Maneuvrier, A., Decker, L. M., Ceyte, H., Fleury, P., & Re-
naud, P. (2020). Presence Promotes Performance on
a Virtual Spatial Cognition Task: Impact of Human
Factors on Virtual Reality Assessment. Frontiers Vir-
tual Real., 1, 571713.
Oberhauser, M., Dreyer, D., Braunstingl, R., & Koglbauer,
I. (2018). What’s Real About Virtual Reality Flight
Simulation?. Aviation Psychology and Applied Hu-
man Factors.
Oberhauser, M., Dreyer, D., Mamessier, S., Convard, T.,
Bandow, D., & Hillebrand, A. (2015, August). Bridg-
ing the gap between desktop research and full flight
simulators for human factors research. In International
Conference on Engineering Psychology and Cognitive
Ergonomics (pp. 460-471). Springer, Cham.
Pallavicini, F., Pepe, A., & Minissi, M. E. (2019). Gaming
in virtual reality: What changes in terms of usability,
emotional response and sense of presence compared
to non-immersive video games?. Simulation & Gam-
ing, 50(2), 136-159.
Pausch, R., Proffitt, D., & Williams, G. (1997, August).
Quantifying immersion in virtual reality. In Proceed-
ings of the 24th annual conference on Computer
graphics and interactive techniques (pp. 13-18).
Peysakhovich, V., Monnier, L., Gornet, M., & Juaneda, S.
(2020). Virtual reality vs. real-life training to learn
checklists for light aircraft. In Eye-Tracking in Avi-
ation. Proceedings of the 1st International Workshop
(ETAVI 2020) (pp. 47-53). ISAE-SUPAERO, Univer-
sit
´
e de Toulouse; Institute of Cartography and Geoin-
formation (IKG), ETH Zurich.
Santiago-Espada, Y., Myer, R. R., Latorella, K. A., & Com-
stock Jr, J. R. (2011). The multi-attribute task bat-
tery ii (matb-ii) software for human performance and
workload research: A user’s guide.
Santos, B. S., Dias, P., Pimentel, A., Baggerman, J. W.,
Ferreira, C., Silva, S., & Madeira, J. (2009). Head-
mounted display versus desktop for 3D navigation in
virtual reality: a user study. Multimedia tools and ap-
plications, 41(1), 161-181.
Singh, R. P., Javaid, M., Kataria, R., Tyagi, M., Haleem,
A., & Suman, R. (2020). Significant applications of
virtual reality for COVID-19 pandemic. Diabetes &
Metabolic Syndrome: Clinical Research & Reviews,
14(4), 661-664.
Sladky, R., Stepniczka, I., Boland, E., Tik, M., Lamm, C.,
Hoffmann, A., & Windischberger, C. (2016). Neuro-
biological differences in mental rotation and instru-
ment interpretation in airline pilots. Scientific reports,
6(1), 1-6.
HUCAPP 2022 - 6th International Conference on Human Computer Interaction Theory and Applications
86